1 Introduction
Monday morning 7:00 AM and Roger was feeling blue. He was slicing bread for, well, for himself. The staled wheat surface offered some response partly turning into crusts below the sharp, serrated knife edge. This being rather normal he did not give the phenomena any greater attention. A rapid thought crossed his mind: -strange how staled this already had gone; the bread was bought fresh on Friday, back when he was still with Julia. He wondered if he had left the bread on the table since it had gone stale already? Had Roger known starch more closely, he might have considered the typical hardening of bread a variant of starch retrogradation in dry conditions. Roger couldn’t be bothered. He continued with breakfast while wondering if Julia would pick up the telephone if he called yet again.
If we do a brief etymological excavation we may find that the word retrograde describes something moving backward in space and/or time, something worsening or going back to an earlier stage or in a general sense something tending to revert to some earlier state. The English word derives from the Latin word rētrōgradiormeaning go back. Here, the Latin prefix retro-means back or backward and denotes a tendency or direction with verbs or nouns of motion. The verb gradioragain denotes going back in time, in past times, before or formerly. In chemistry again, retrogradation refers to the general reversion of starch to a more ordered structure upon cooling. This occurs basically in natural starches to some degree. Principally this is a type of natural reassociation (reorganization) of once heated (disorganized), hydrated and solubilized starch molecular chains. This reversion occurs gradually and takes place in both dry and wet conditions. Why is it so then? Let’s have a look at the case relevant in papermaking applications.
2 Native organization
Starch, the major energy storage polysaccharide of higher plants, is a polymeric mixture of essentially linear and branched α-D-glucan molecules. Natively starch is formed as granules, these are partially crystalline, and their morphology, chemical composition and super molecular structure are characteristic to each plant species. Starch owes much of its functionality to two major high-molecular-weight carbohydrate components, amylose and amylopectin, as well as to the physical organization of these macromolecules into granular structures. To us, starch is also an important component in paper and board grades.
The linear amylose consists of a-(1,4)-linked ‘sugar units’ (D-glucopyranosyl). The molecules also havea-(1,6)-linkages providing branched structures. The degree of polymerization ranges from 500 to 6,000 glucose units implying molecular weights (MW) from 8×104 to 106Dalton. The highly branched amylopectin contains 30,000–3,000,000 glucose units and, therefore, it has a considerably higher MW(107–109) than the linear amylose. The branched amylopectin arrangement together with strands of amylose form double-helical structures. The highly branched amylopectin structures are partly responsible for the ingenious granular nature of starches. Natively, the starch granules are built of concentrically alternating amorphous and crystalline zones, so-called growth rings, and are kind of analogous to the structure of an onion. Each zone, or ring, contains numerous smaller blocklets of well-arranged super-helices. These again are built up by lamellas of double-helices consisting of parallel, helically arranged amylose molecular chains branched by the amylopectin. It is due to its hierarchic micro-level structure, molecular conformation and the easily hydrolysable molecular linkages that starch makes such a compact form of chemical energy in nature as the helical backbone allows for formation of tight, granular shapes in native plants.
3 Hydrothermal treatment – disorder by heat
The common way of preparing starch in industrial scale today is hydrothermal by so-called jet-cooking. This is a rapid and continuous process for preparing granular starch as dispersions for various applications, as made in paper industry. Under excess steam conditions, the high-temperature and high-shear conditions resulting from passing high-pressure steam (2-4 bar overpressure, depending on temperature) through the hydro-heater disrupts the granular structures. This occurs upon dissolution of both amylose and amylopectin components while also reducing the starch molecular weight. The thermal and mechanical high-shear energy induced to the water-solubilizing starch at elevated temperature is a key reason behind the losses in molar weight. During jet-cooking the granules initially swell and become gelatinized. All this implies breakage of hydrogen bonds between starch chains within the granular structure. The disarrangement occurs due to hydration by high-energy water molecules. At first, the amorphous regions become wet and start to swell, which we observe as an increasing viscosity passing a peak value. Subsequent thermal energy makes a starch paste disrupting the granule while leaching the amylose as the starch chains are loosening up their ordered structures. Secondly, the crystal regions are swelling and begin to open up, unwinding the starch chains whilst successively becoming more and more solubilized with the hot water. One mechanism proposal has been that the aligned (crystalline) amylose regions tend to take up water forming local ‘gel-balls’. These changes are collectively referred to as starch gelatinization and are accompanied by the loss of characteristic birefringence of intact granules. At some point the starch molecules helical structures become sequentially more released and here the viscosity begins to drop and is gradually stabilized to some level. At this point the starch granules have been disrupted to a maximum level and are dispersed at maximum level. The jet-cooking is an efficient hydrogen debonder: within 90 s at temperatures of 120–140°C, depending on the starch grade, we have a smooth pumpable and fluid liquid of dissolved starch where most of the starch chains are released from their original ordered, granular structures. The induced thermal and mechanical energies disorganize the starch both in a physical and chemical way. The latter will to some degree result in splitting of starch chains which is practically inevitable when processing large quantities and is not considered any problem at any stage in today’s reality. The functionality of a starch-based product is a more central point.
4 Starch retrogradation – reorganization
When starch has been gelatinized or cooked into viscous starch paste and begin to cool down the disaggregated starch chains tend to begin an immediate reassociation by reforming hydrogen bonds. From the carbohydrate chains’ point of view, it is energetically more favorable trying to transform back into their previous well-order hydrogen-bonded structure. This could even be considered a natural memory effect, also exhibited by other native structures e.g.cellulose that also tend to remember its previous structural state, however at specific conditions. The freed starch molecules gradually begin to network by forming local, partially ordered structures. The reorganized structures induced in a pasted starch differ from those observed in native starch granules and differ depending on starch origin.
Hence, starch retrogradation is a process in which disaggregated amylose and amylopectin chains in a gelatinized, smooth starch paste tend to reassociate to form more ordered structures. Retrogradation is an ongoing process, which initially involves a more rapid reassembly of amylose molecules followed by a slow recrystallization of amylopectin molecules.
The typical retrogradation of starch is usually accompanied by a series of physical changes such as increased viscosity and turbidity of pastes, gel formation, exudation of water (syneresis).
5 High temperature retrogradation
The previous case of network formation takes places as the starch is cooling down. If the starch solution reside in a higher temperature region we may experience other types of phenomena, however arising from the same restructuration tendency. Here, a crystallization process starts from spontaneously formed complex or nuclei from which crystallites begin to grow in a spherical arrangement. It has been shown that so-called spherocrystals are formed from a cooked starch paste when stored at 75–95 °C. These spherical shapes consist of well-arranged helical inclusion complexes of amylose and native lipids, that are originally present in the starch granule. The authors termed these crystallites high-temperature retrogradation products since a high processing temperature in the jet cooker and a high storage temperature were required for their formation. Recent work has looked more into detail on how these structures are formed. A key factor behind the spherical particles is the presence of suitable fats.
5.1 Fat or lipids in starch
As you may know, starch granules usually contain some built-in fats, i.e.endogenous lipids that are, variant in content (1–8%) and composition between plants. Lipids associated with isolated cereal starch granules have been found to occur on the surface as well as inside the granule. The surface lipids are mainly triglycerides, followed by free fatty acids, glycolipids and phospholipids. They include those derived from the starch-generating amyloplastic cell membranes as well as those from non-starch sources. The internal lipids of cereal starches are predominantly monoacyl lipids, with the major components being phosphor-containing lipids (phospholipids or their digested variants lysophopholipds) and free fatty acids in e.g.wheat, barley and rye. The major fatty acid in neutral lipid fractions is palmitic (16:0) in wheat, corn, potato and cassava, and linoleic (18:2) in rice and lentil, while in glycolipid fractions it is linoleic in cereal starches and palmitic in cassava. However, in phospholipid fractions all starches have palmitic as their major fatty acid.
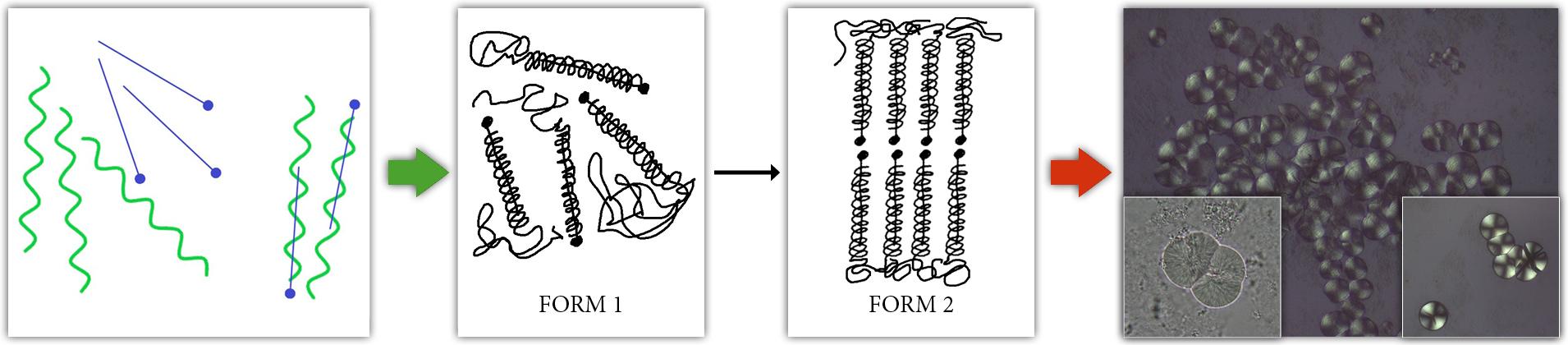
Cereals are considered to contain simple, complex and secondary fats, or lipids. Depending on cereal species, an average lipid contents of 1.7–7% in the grains are present. Oats are rich in lipids (6–8%) in contrast to wheat and rye (1.7–2%). Cereal lipids have similar fatty acid compositions, in which linoleic acid reaches contents of 39–69%, while oleic acid and palmitic acid make up 11–36% and 18–28%, respectively.
6 Lipid-amylose complex formation
Starch lipids are primarily composed of lysophospholipids, which form inclusion complexes with amylose helices already in native starch. To illustrate, these complexes are comparable to a pin (with a head) residing inside a helical structure, akin corkscrew or fusilli pasta.
In barley, wheat, and rye starches, the starch lipids are almost exclusively lysophospholipids (LPL), which have been shown to exist as amylose-lipid inclusion complexes in native starch granules rather than being formed during starch gelatinization. Heating barley starch-water suspensions at >94–100°C induces dissociation of the amylose-lipid (AML) complex. The dissociation of the AML complex is a reversible phenomenon, and reformation of the complex occurs during cooling.
Cereal starches contain only around 1% of lipid and therefore it is important to note that the lipid fraction within starch granules is insufficient to saturate the amylose fraction and hence form fully saturated amylose–lipid complexes. Amylose is referred to as lipid-free amylose or lipid-complexed amylose which may be differentiated by various analytical methods. These forms may have specific locations within starch granules. Formation of complexes between amylose and lipids greatly affects starch properties. This occurs during heat/moisture treatments, especially during gelatinization of starches with naturally containing lipids. Both naturally occurring, and heat-formed complexes display specific properties such as a decrease in amylose solubility and swelling, an increase in gelatinization temperatures and prevention of amylose-leaching from starch granules.
7 Conclusion
To sum up, it can be said that starch retrogradation is a natural phenomenon driven by entropy changes while also a quite multi-faceted topic where several variables are identified. A closer look on the phenomena reveals that the main factors behind this reorganization – we are dealing with a type of natural molecular self-assembly – are caused by the structure, design and interactions of starch molecules. To pin-point the topic it is all about hydrogen bonding, these are broken by thermal energy and only so-to-say want to reform due to energetic reasons. Nevertheless, several tools can be found to characterize the phenomena, even down in detail. Ways to reduce and even control the re-assembly are available, even in industrial scale. A central issue in the mechanism behind starch high-temperature reorganization is the presence of lipid molecular species. These tend to form the initial complexes that trigger the reorganization – small disturbances like these are a central key in any molecular-level re-assembly.
These phenomena are occasionally encountered in papermaking applications and may give rise to peculiar issues like dry paper dusting, filter blockages and strange deposits. Nevertheless, these issues are avoidable when we know what we are dealing with and know how to prevent the issues by proper process control.
Getting back to the issue with Roger. We thank him for this weakish metaphor and offer the following piece of advice; Getting back with your ex is like reheating a McDonald’s meal.
[Tom Lundin D.Sc. (Tech.) Senior Expert (Sales and R&D) & Shaoxia Wang D.Sc. (Tech.) Independent Specialist]
8 Literature
- https://latinlexicon.org/
- Starch: Chemistry and Technology Eds.: BeMiller J. and Whistler R. 2009, Academic Press
- https://www.foodscience-avenue.com/2014/11/what-is-retrogradation.html
- https://www.etymonline.com/word/retrograde
- http://www1.lsbu.ac.uk/water/starch.html
- Donald (2004) A. M. Donald, Understanding Starch Structure and Functionality, Chapter 5 in Starch in Food: Structure, Function and Applicationsby Ann-Charlotte Eliasson, CRC Press
- Blazek, J. (2008) Role of amylose in structure-function relationship in starches from Australian wheat varieties, PhD-thesis, University of Sydney, Australia
- Gobbetti and M. Gänzle (eds.) (2013) Handbook on Sourdough Biotechnology, 11, Springer Science+Business Media
- Rosicka-Kaczmarek,J.etal (2015) 2.6 Composition and functional properties of lipid components from selected cereal grains. in: Plant Lipids Science, Technology, Nutritional Value and Benefits to Human Health, (Eds. Grażyna Budryn and Dorota Żyżelewicz)
- Byars, J.A. (2003) The effect of cooling conditions on jet-cooked normal corn starch dispersions. Carbohydrate Polymers 54 (2003) 321–326
- Shamekh, S. (1998) Enzymatic Hydrolysis of Barley Starch Lipids. Cereal Chem. 75(5):624–628
- Bhosale and Ziegler (2010) Preparation of spherulites from amylose–palmitic acid complexes. Carbohydrate Polymers 80 (2010) 53–64
- Creek (2007) nanoscale self-assembly of starch: phase relations, formation, and structure. PhD-thesis, Pennsylvania State University, USA
- Lay May, U.V. (2011) Effect of starch fractions on spherulite formation and microstructure. Carbohydrate Polymers 83 (2011) 1757–1765
- http://www.chemistryexplained.com/Ny-Pi/Phospholipids.html